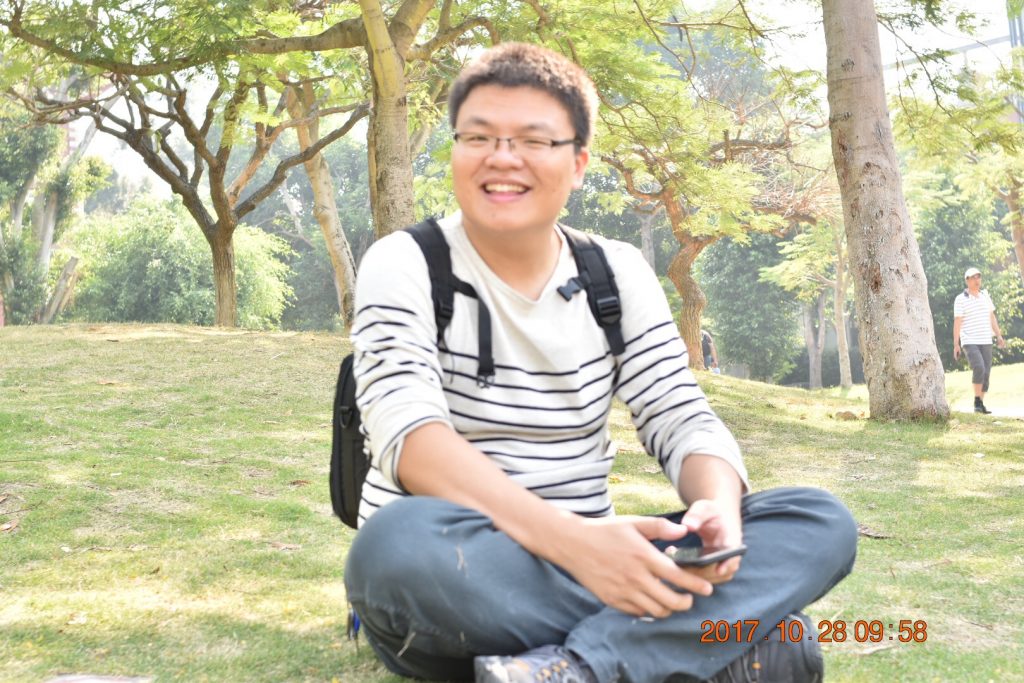
By Erika Raven
We met with Lin Chen and Jiadi Xu from the Kennedy Krieger Research Institute at Johns Hopkins University to discuss their paper, “Separating fast and slow exchange transfer and magnetization transfer using off-resonance variable-delay multiple-pulse (VDMP) MRI”. First of all, a little about the two authors: Lin obtained his PhD in 2017 from Xiamen University in China, then traveled ‘round the world to research quantification methods for chemical exchange saturation transfer (CEST) MRI. Jiadi has a background in solid-state and solution NMR. He works to detect all types of exchangeable protons using MRI and tissue modeling. Their paper attempts to separate and quantify CEST signals based on exchange rates. This is different from a typical CEST experiment that targets a spectral frequency associated with a specific metabolite (e.g. creatine), or filters out “unwanted” contributions, such as those from macromolecules using their unique line-shapes. The VDMP approach can detect all contributions, as long as they exchange, and is not even limited to metabolites.
MRMH: Can you briefly outline your technique?
Lin: Many existing CEST methods are good at detecting clear peaks in Z-spectra. But in the presence of overlapping CEST peaks, they become invalid. The proposed method provides a new dimension for separating and quantifying CEST signals in Z-spectra based on the exchange rates. Here, we divided these into two categories: fast (>1 KHz) and slow exchange rates. The contributions of fast and slow exchanging components can be obtained by their characteristic VDMP build-up patterns.
MRMH: Did you have any ‘ah-ha!’ moments when developing the technique, or draw inspiration from previous work?
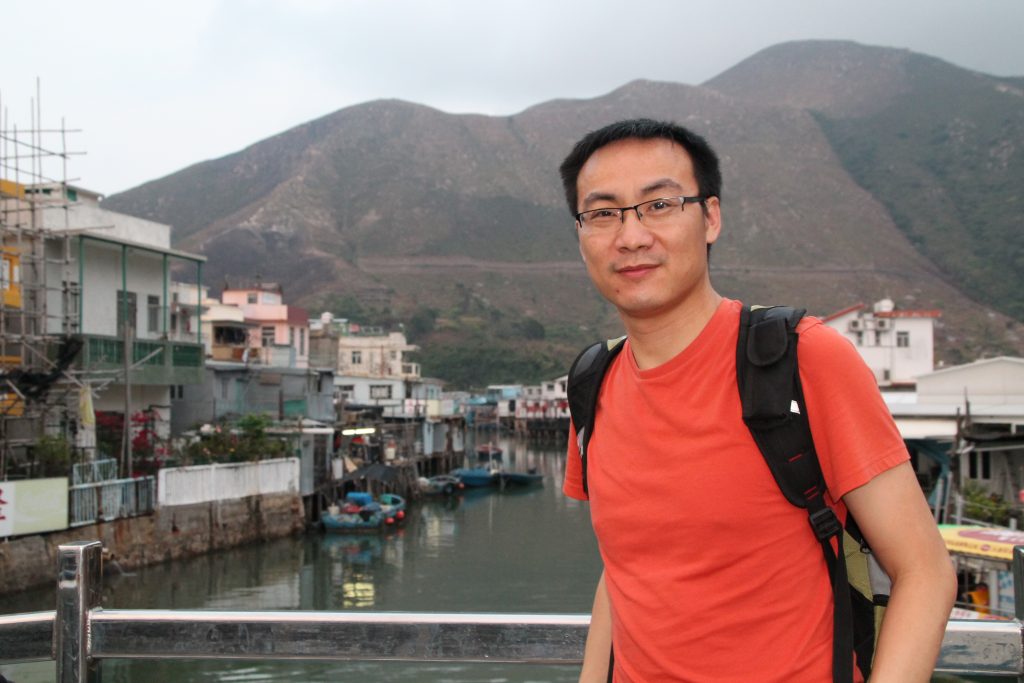
Jiadi: This technique borrows the idea of ‘mixing time’ from a very important NMR method called NOESY (nuclear Overhauser effect spectroscopy). In NOESY, mixing time reflects changes based on the distance between protons and with this you could finally calculate a structure from it. Actually, that was how Kurt Wüthrich and Richard Ernst got their Nobel prize for research in NMR.
When I began working on CEST, people seemed to just be using the mixing time as a duty cycle, which confused me for a while. Since I come from an NMR background, I could see that the mixing time was very similar to the NOESY study, and could therefore play an important role, beyond just adding to the duty cycle. That was actually the ‘ah-ha!’ moment when developing the VDMP method. Of course, during its development, I drew considerable inspiration from discussions with my mentor Peter van Zijl, and also my colleague, Nirbhay Yadav.
MRMH: Z-spectra seem like a murky place. Can you break down the sources of magnetization we’re dealing with here?
Lin: Z-spectra are the outcomes of CEST experiments. To generate them, a saturation pulse is first applied to saturate the magnetization of the protons present in metabolites. Then, thanks to the action of exchangeable protons, this magnetization will transfer to water and reduce the water signals. This means we can detect changes from water and use this information to quantify the various proteins and metabolites.
Jiadi:: The negative part of Z-spectra contains aliphatic protons from both lipids and proteins. The positive part is much more complicated. For example, there are amide protons from the backbone of the amino acids in proteins. Also, some amino acids in proteins, such as arginine, asparagine and serine, have additional exchangeable protons in their side chains. Furthermore, some metabolites contain amine and hydroxyl exchangeable protons. All the exchanging protons are crowded within a narrow range, from -5 to 5 ppm.
MRMH: With so many metabolites in the spectral mix, how do we decide whether to keep or ignore certain signals?
Jiadi: Separating the individual contributions of metabolites is undoubtedly challenging, and it is possible only for certain special compounds, such as creatine and phosphocreatine, which show sharp peaks in Z-spectra. Hence, we try to separate the fast- and slow-exchanging compounds. In the current study, we did not try to suppress any contribution, just to separate and quantify them.
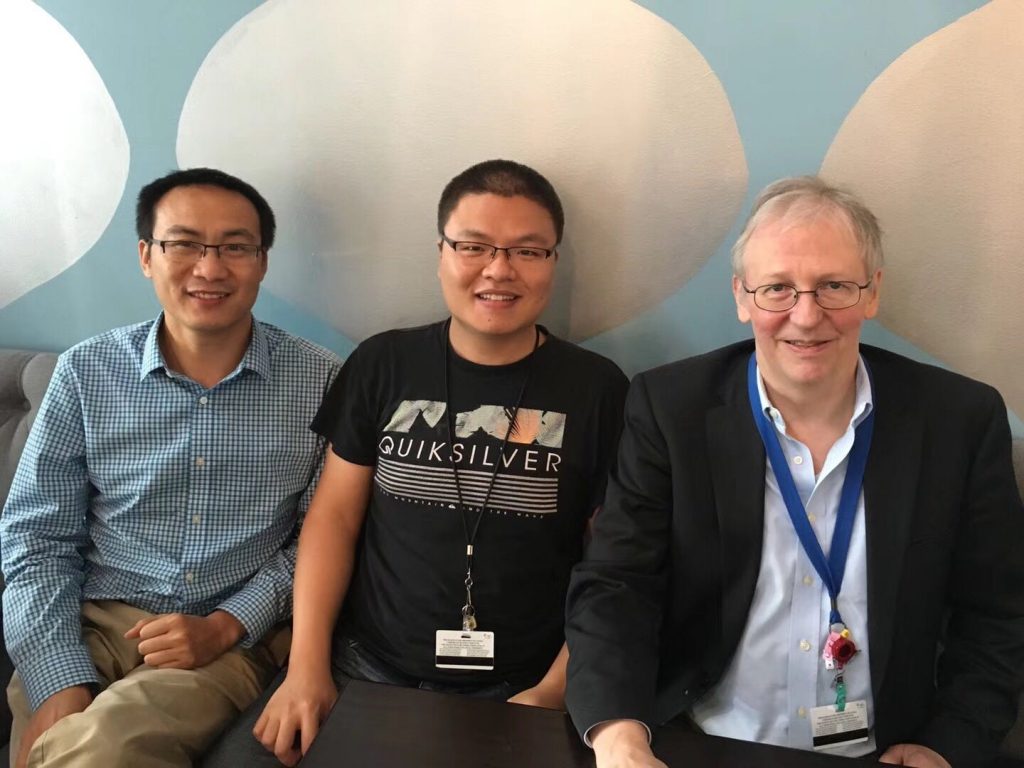
MRMH: What experimental conditions might shift fast and slow exchange?
Jiadi: Fast and slow exchange rates are separable by varying the mixing time of the pulse train. The ratio between them can be controlled through the saturation power of the pulse. If we use weak power, then we mainly see the slow-exchanging protons, such as amide and aliphatic protons. If we use high power, then the fast-exchanging protons will be stronger, but we will see strong magnetization transfer (MT), too, since they also contain slow-exchanging process.
Lin: Besides the experimental parameters, there are other factors, such as the temperature and pH value that will also shift fast and slow exchange.
MRMH: Any caveats for those looking to separate exchange components in their signals?
Jiadi: It is important to bear in mind that this is a transient method and that it uses a build-up of CEST contrast. Hence, you need to keep the pulse number relatively small. Also, the saturation power cannot be too strong, otherwise the build-up will be dominated by MT. Also, direct saturation of water will shorten the build-up process and reduce the build-up signal with strong power.
MRMH: What are the future directions for this technique?
Jiadi: Actually, the method has already been used in many studies. One involved tuning the mixing time to remove MT and get pure amine and aliphatic peaks. Also, the fast-exchange component is sensitive to pH, and can be used for pH mapping. We demonstrated this on a stroke model in collaboration with Nauder Faraday and Kathryn Schunke. This technique is not specific to CEST; it works for any exchange process in MRI, such as arterial spin labeling.